Landmark trials with gene-edited barley have demonstrated the strong interaction between cultivated crops and soil microbes that stretches back over 460M years. CPM visits the trial site to explore the implications.
“There is so much to learn about the cross-talk between plants and microbes.”
By Tom Allen-Stevens
The seemingly stunted, haphazard growth of the barley in the trial plots could belie the significance of what lies below. For Dr Tom Thirkell, who’s moving through the small, square-metre plots of barley, just the fact they’re growing at all is already a landmark achievement.
“Every growth stage we reach is a milestone in itself,” he comments.
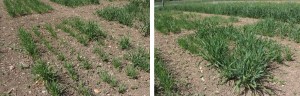
In the GE lines of Golden Promise barley (left), a gene that allows plants and microbes to interact has been knocked out while this has been overexpressed in GM lines.
These are the first gene-edited (GE) barley trials in the UK. Tom and colleagues at the Crop Science Centre, Cambridge, have used the precision-breeding technique to explore the properties of a nodulation signalling pathway gene (NSP2). This allows plants and microbes to interact and it’s been knocked out of GE lines of Golden Promise barley.
These are grown in plots in the field alongside GM lines in which the gene has been overexpressed. It’s part of a long-term project to find out if cereals can be developed with the ability, like legumes, to fix their own nitrogen from the atmosphere.
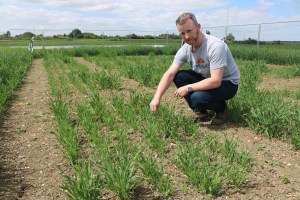
Tom Thirkell’s real interest lies in the arbuscular mycorrhizal fungi and making sure the plants are getting the most benefit from the fungi as possible.
Tom’s real interest, however, lies in the arbuscular mycorrhizal fungi (AMF) that threads its way through the soil beneath his feet, or more rather, the relationship this has with the barley plants. “Mycorrhizal fungi are found in virtually all farm soils, forming this symbiosis with our crops. The challenge now is making sure the plants are getting the most benefit from the fungi as possible. Farmers I meet are also enthusiastic about beneficial soil organisms, and with good cause.
“There is so much to learn about the cross-talk between plants and microbes – what tells a plant to block pathogens, or allows another organism to colonise. If we can understand and influence these signalling pathways, the potential to reduce reliance on synthetic inputs is immense,” he says.
So that’s been the point of the trial – testing the effect of the gene believed to be at the heart of the relationship between plants and AMF, and then exploring closely the effect in a field situation.
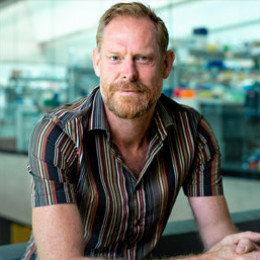
The symbiosis between plants and fungi started around 460M years ago, says Giles Oldroyd.
It’s a relationship that’s one of the oldest and most successful in existence, but it’s one of the least understood and is considerably under-utilised, according to Professor Giles Oldroyd, who leads the research at Crop Science Centre. It could even hold the key to feeding the world and reversing the effects of climate change, he says.
“There’s a beautiful fossil record that shows the symbiosis started around 460M years ago. It began when plants first moved onto the land and had no roots, so built a relationship with fungi in the soil.”
AMF are remarkably abundant, accounting for 5-50% of the microbial biomass in agricultural soils. The network of hyphae they form can be equally astonishing, with as much as 100m in just one gram of grassland soil. These hyphae lack regular cross walls, allowing the rapid passage of materials through the network. This massively increases a plant’s effective root length, depth, surface area and soil volume from which it can draw nutrients and other resources.
The fungi also grow inside plant roots, and that’s how the special relationship has developed. Where plant and fungal tissues meet, the fungi form special structures known as arbuscules. These have a large surface area that allows nutrient exchange between the partners.
Around 70% of all plant species have retained the symbiosis – brassicas represent a rare crop type that has evolved a different method of extracting nutrients from the soil, and have long since lost the association with AMF. For other crops, notably cereals, it has evolved into a complex system within plants of signalling pathways and the expression of genes that turn these on and off, producing a chemical, strigolactone, that soil microbes can detect.
“We know that NSP1 and NSP2 within plants are central to this process,” explains Giles. “If a plant needs nutrients, these genes are expressed which trigger a chain of signals. Phosphate and nitrogen, for example, can be taken up from the soil through this symbiosis.”
But the genes are only expressed when the plant goes short of nutrients – synthetic inputs make the system redundant, so AMF tends to be more abundant in organic and low-input systems. Soil disturbance disrupts an AMF network, so its growth is most advanced and complex in no-tilled soils, especially permanent pasture, field edges and woodland.
So how does this relate to nitrogen fixation? “This is an ability that evolved later – around 100M years ago – but utilises some of the same signalling pathways,” Giles continues. “The interesting aspect is that there are many associations of plants with fungi, but only legumes have evolved this symbiosis with nitrogen-fixing bacteria.”
The ultimate aim for Giles and his team is to pinpoint what it is that allowed this to happen in legumes and recreate it within cereals. Initial work in this area started around 25 years ago with work on a model legume, Medicago truncatula, exploring the genetics that allow it to engage with nitrogen-fixing bacteria.
“We identified the genes responsible and created mutant plants in which their ability was knocked out. The result was very severe and they not only lost the interaction with nitrogen-fixing bacteria, the mutation almost completely abolished the association with AMF.”
What the researchers learned from this was profound. “For legumes, evolving the capability to engage with nitrogen-fixing bacteria was not something totally novel. What they did was to build on a pre-existing framework that already engaged with AMF. So it follows that there’s a genetic framework present in most plant species that can be adapted to do the same – the symbiosis signalling pathway is already there,” notes Giles.
“We understand reasonably well the nature of this capability, and that’s what we’re aiming to engineer into cereals. But it remains a big leap to actually build the ability for cereals to fix their own nitrogen, and we’re not there yet.”
In the meantime, the team is exploring further the signalling pathways related to NSP2. Barley is the chosen cereal as it’s a diploid – less complicated to alter genetically than hexaploid wheat. There are four lines in the trial with the unaltered Golden Promise control – two are GE lines in which symbiosis signalling genes have been disabled.
Then there’s a GM line that has DNA from M. truncatula which allows the barley plant to overexpress NSP2. The fifth line has native barley DNA inserted in, such that the response of NSP2 is no longer triggered by nutrient starvation, but always active. This is a method known as cisgenesis, explains Giles, using DNA from within the same species, but classed as GM as it’s a genetic change that couldn’t occur naturally.
“It’s very easy to knock out a gene function with GE, but much harder to improve it through GE, although our capabilities in this area are progressing,” he says.
But whether GM or GE, nitrogen fixation or feeding the AMF, there’s a carbon trade-off – around 20-30% of carbon captured by a plant can be transferred to the microbes in the soil. “Nitrogen fixation is a very energy-demanding process for a plant,” adds Giles.
“While you can probably enhance photosynthetic efficiency to compensate, the target market for nitrogen-fixing cereals is not initially here in the UK, where you’re already achieving relatively high levels of a plant’s potential productivity using synthetic inputs. The advantage is for the smallholder in Africa, who currently gets about 15% of its potential because they have just bare soil to work with, and no added nitrogen, whether organic or inorganic.”
Giles predicts the balance of priorities in wealthier nations is bound to change, favouring nitrogen-fixing cereals, as farming moves away from synthetic inputs towards more sustainable practices. But developing the relationship with AMF may hold far more promise for the UK cereal farmer.
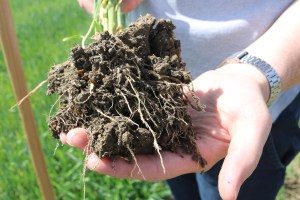
Fungal carbon is very long lived in the soil, so AMF has great potential as a way to sequester carbon.
“AMF are carbon auxotrophs – the only way the fungi can get carbon is from the host plant. That comes in from photosynthesis – the plant fixes CO₂ from the atmosphere, shuffles it down into the root and then feeds it to the AMF which then builds a hugely complex fungal network in the soil.”
Currently this process is regulated by the plant’s perception of its environment, and when it’s optimal to engage with the AMF, which is currently only when it’s near starvation, he notes. “But what if you adapt this process so it’s optimised for agriculture? That’s what we’re doing right now with the genetics were developing.”
The potential advantages of cereal crops adapted and tuned to actively feed the soil go far beyond improvements to soil health and fertility and could outstrip the nutrient cost savings, believes Giles.
“Fungal carbon is very long lived in the soil – much longer than plant carbon. So as a way to sequester carbon in your soil, this is phenomenal. If farmers are going to be paid to sequester carbon, then this is the mechanism to do so,” concludes Giles.
Field trials pave way to farmer involvement
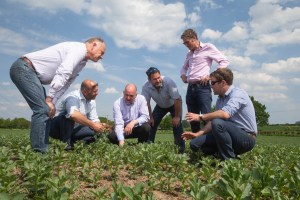
The aim of the farmer-led trial, coordinated by BOFIN, is to get an accurate picture of the AMF levels in the soil for each of 30 samples taken from across the UK.
The first year’s trials were taken successfully to harvest this summer, and the team has been painstakingly trawling through the data and studying the properties of the material the plots have yielded.
Now a new, farmer-led project aims to measure the level of AMF in UK arable soils and explore this interaction further.
“We’ve yet to assess all the data, but initial analysis from the trials suggests we’re seeing comparable behaviours in the field to those we’ve seen in the laboratory. It shows we can have strong control over when plants engage with these beneficial fungi,” reports Tom.
The field trials will be repeated for a further two years to confirm findings. There are also plans to look at other barley varieties – Golden Promise has been used because much of its genome has been mapped and characterised.
“We’re screening other varieties, including those on the AHDB Recommended List – we know the response to AMF varies so we want to explore this,” notes Tom. “The rewards may be substantial – AMF can provide a crop with all of its phosphate requirement and a large proportion of its N, sometimes more effectively than plant roots alone. There’s also the influence of P-solubilising bacteria, which is another symbiosis we’re keen to explore.
“But increasing AMF colonisation doesn’t always benefit the symbiosis and there may even be a level at which parasitic behaviour occurs. We simply don’t know and it’s why the field trials are important – these are interactions you can’t understand if you only study them in the lab or the greenhouse.
“What’s more, we know very little about the actual amount of this fascinating fungi we have in arable soils, how this varies and how it’s influenced by management practice,” adds Tom.
So this is the focus of a new farmer-led project, co-ordinated by BOFIN (British On Farm Innovation Network). “We plan to take plant samples with roots intact from around 30 first wheat crops in May or June this year,” he explains.
BOFIN is looking for around 30 farmers to join the Soil Squad – a group who will have their plant roots analysed for AMF activity. BOFIN and CSC will then work with the Soil Squad to ensure the samples are taken correctly and give an accurate picture of the AMF levels for each of the samples taken.
“You can quantify and DNA sequence AMF relatively easily with the right lab and equipment from the plant roots alone. It’s not just the quantity that’s important for the symbiosis, but the agronomy too and we’re keen to understand more about that. We’re hoping to deliver the results at the Groundswell event in June.”
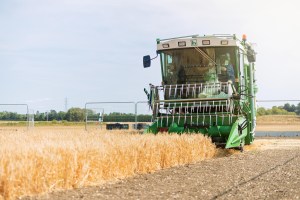
Following a successful harvest of the first year of trials, there are now opportunities for farmer involvement to complement the research. (Photo: CSC)
“This is the first time UK arable soils have been benchmarked in this way, and I’m really excited about what we’ll find,” enthuses Tom.
In future, there may also be an opportunity for farmers to actually test GE lines. Since March this year, precision-bred organisms can now be included in field trials in the UK without the restrictive GM regulations that currently govern how GE crops are grown in field trials across the EU.
Giles indicates he’s particularly keen to work with regenerative agriculture growers. “I don’t think the soil we’re working with here on the NIAB farm necessarily makes the most of the differences between the GE lines with the NSP2 gene knocked out and those where the expression is enhanced. Similar trials with maize have shown a 30% yield difference.
“I suspect doing the trials with a regen ag farmer who understands the role of AMF and has nurtured levels in their soil would show a much stronger effect. What’s more, we have just developed a true GE line that overexpresses NSP2, so I’d be super excited to test that in a real farm situation. The only barrier that’s been in our way until now has been the GM regulation,” he notes.
Anyone can apply to join the Soil Squad – the aim this year is to gather samples from a spread of farming systems and cultivation approaches. An introductory webinar on Thursday 16 February provides details for those interested in being involved. More at www.bofin.org.uk.
This article was taken from the latest issue of CPM. For more articles like this, subscribe here.