Two decades of pioneering plant science in the UK have brought into the field staggering advances in genetics. Now it’s time for growers to get involved. CPM took a tour to open a window on a world of wonders.
It’s about making use of a technology that can accelerate how we bring our discoveries to the field, and the shorter timescales will bring in farmers.
By Tom Allen-Stevens
They look like little more than knots of root and shoot matter held in place in the agar of a petri dish as Dr Sadiye Hayta holds them up towards the web cam for Prof Cristobal Uauy to view. But these represent something of a breakthrough for the team at the John Innes Centre (JIC) working on Designing Future Wheat (DFW), and a virtual high five passes between the two colleagues.
“What we’re looking to establish is genotype independence,” explains Cristobal, who’s joined the meeting via Zoom web conference. “We’ve been working with a genetic trait of Triticum polonicum, a durum wheat that has unusually long glumes and very long grains. We’re aiming to introduce the trait into the hexaploid varieties Paragon and Fielder and another durum wheat, Kronos, through gene-editing.”
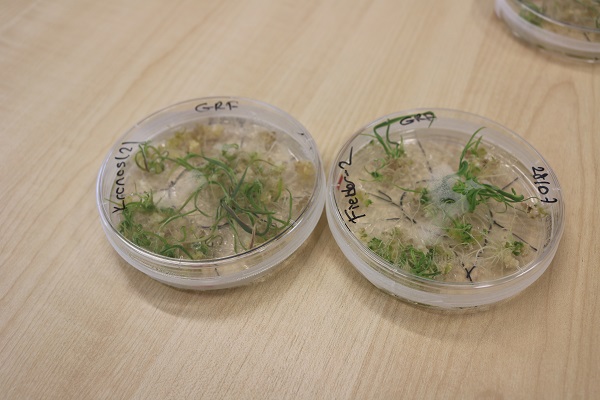
Scientists at JIC are looking to establish genotype independence, introducing novel traits directly into elite lines.
This process is currently genotype dependent which limits the ability of scientists to use the new plant-breeding technique (NPBT) to introduce new traits into commercial, elite lines, particularly those that no longer exist in modern varieties. “We’ve been working on a new technique developed by colleagues at University of California Davis for a year and a half, and this represents the first sign of success, which could be revolutionary – it’ll mean we can turn around near isogenic lines (NILs) of elite varieties in just 2-3 years,” he enthuses.
This is currently a process that takes at least eight years through conventional plant breeding, even when using modern gene markers and doubled haploid techniques, he explains. Once a trait is identified, the wheat type that has it is crossed with an elite line that would benefit from it. Successive generations are then crossed and screened to sift out hundreds of other undesirable traits that have come over in the cross, to reach the NIL – the commercial line with just the new introduction.
“It can take 10-15 years to bring in more unusual and exotic traits, particularly if it comes from landrace or wild varieties,” Cristobal continues. “It’s a process that’s not viable for traits that are less commercially valuable which is why current UK wheat varieties are bred from a very narrow gene pool. Breeders are simply shuffling the pack, but DFW aims to bring new cards into play, which could be transformative.”
DFW is a BBSRC-funded programme spanning eight UK research institutes and universities. A five-year series of four work packages that started in 2017, it follows on from the Wheat Institute Strategic Programme (WISP) and involves more than 25 groups of scientists across Rothamsted Research, JIC and Earlham Institute, with additional contributions from NIAB, the European Bioinformatics Institute (EBI), and the Universities of Bristol and Nottingham. Key features of DFW are that all the knowledge is made publicly available and UK commercial breeders are involved from the outset.
Driving forward this public-funded search for the new wheat germplasm containing the next generation of key traits is the projection that the world will need to produce 60% more wheat by 2050 to meet global demand. But yields in the UK have plateaued and it takes 15-20 years for current research to improve wheat varieties grown in farmers’ fields.
That’s encouraged a deep delve into the very fundamentals of the wheat genome – research credited as unique to the UK, and now accelerating as new techniques evolve. Some of the world’s foremost plant scientists have explored landrace varieties, germplasm previously never included in wheat-breeding programmes, and even returned to the original cross that 10,000 years ago formed today’s hexaploid wheat (Triticum aestivum) from tetraploid emmer wheat and goat grass.
Now, some 12 years after WISP first started, the first of the most promising finds from this pre-breeding community are making their way from the lab to the field. From tiny first generation plots formed from one ear of wheat to replicated field trials of NILs, covering several square metres, novel material of every shape and form can be found in the fields of research institutes and universities across the UK and even in commercial breeders’ plots among their elite lines.
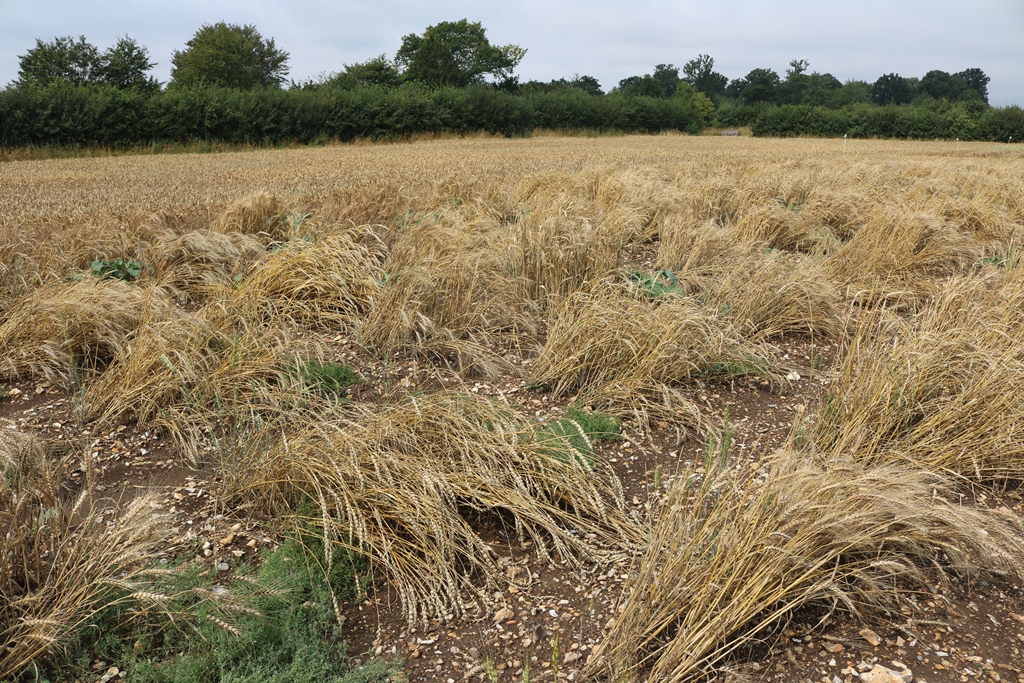
Growers get the chance to grow slug-resistant wheat, currently in pre-breeding trials. Also NIAB synthetic wheats and healthier flour.
“Some of the most exciting traits have been found in the Watkins collection,” notes crop physiologist at Rothamsted Research Andrew Riche. He’s been involved in some of the high throughput phenotyping – spotting the varietal differences in the field that have come about from the thousands of crosses made. He uses drones to assess traits such as nitrogen use efficiency, crop development and biomass yield as well as Rothamsted’s impressive Field Scanalyzer. This comprises a gantry with a motorised measuring platform festooned with sensors that monitor crops to a high degree of resolution and reproducibility.
“A E Watkins was a British botanist who collected around 1000 wheat cultivars from around the world during the 1930s, now held at JIC,” he explains. “These have been genotyped and there are 120 lines that represent the overall genetic diversity of the collection – far greater than the diversity currently used by European and UK breeders.”
These have been back-crossed into Paragon and, along with the many other novel crosses coming through from the DFW partners, the resulting NILs enter the Academic Toolkit. This operates a bit like a Recommended List for breeders, says Andrew. “Lines are selected each year to go forward to the Breeders Toolkit where material is made available to breeders along with genetic markers.”
There are 16 lines going forward from the 2020 harvest of the trial plots, with this the third year the system has been operating. “There’s talk of stepping up the programme, and it’s not just about yield – there are some very interesting disease and pest resistances being studied as well as grain quality and nutritional benefits, such as improved zinc and iron content. The involvement of breeders has been pivotal in bringing this material into the field,” he notes.
But this move to the field is posing a problem. Some of the material has been developed using NBPTs, specifically gene-editing, which have been classed as genetically modified organisms (GMOs) by the EU. This restricts their use and how they’re released to the environment. Most other nations, including the US and China, view NPBTs as conventional, however, and most UK plant scientists agree. Defra has always maintained that “gene-edited organisms should not be regulated as GMOs if the changes to their DNA could have occurred naturally or through traditional breeding methods”.
The Government’s now announced there’ll be a consultation this autumn on whether to diverge from EU policy on gene-editing and much may hinge on the feelings of UK growers if NBPTs are ever to be planted in their fields. But a recent survey undertaken by the Gene-Editing for Environmental and Crop Improvement initiative showed as much as a third of even the more progressive arable farmers feel they don’t know enough about NBPTs to have an informed opinion.
And that’s why these knots of promising germplasm hang in the balance. So too does much of the research that sits behind them, captured into wheat plants of every description in JIC’s light rooms and glasshouses at Norwich Science Park. The institute is at the forefront of wheat research globally and one of its landmark successes has been the development of a GM wheat with a natural high-iron content in its endosperm.
“The aim is to address iron deficiency anaemia, a significant global health problem,” explains senior geneticist Prof Wendy Harwood. “Tests on the newly developed variety grown for the first time in the field last year showed that the grain contained double the amount of iron in its white flour fraction.”
She points to where the trial took place, inside a high-fenced, locked enclosure. This is one of only two field locations in the UK specially adapted for ensuring the controversial field trials of GMOs can continue within the current regulations and without disturbance by anti-GM activists.
“We don’t get much bother from activists these days, but it was COVID-19 that upset trials due to take place this year. Lockdown occurred just as colleagues were about to set out the trial, although we have continued with the research under glass.”
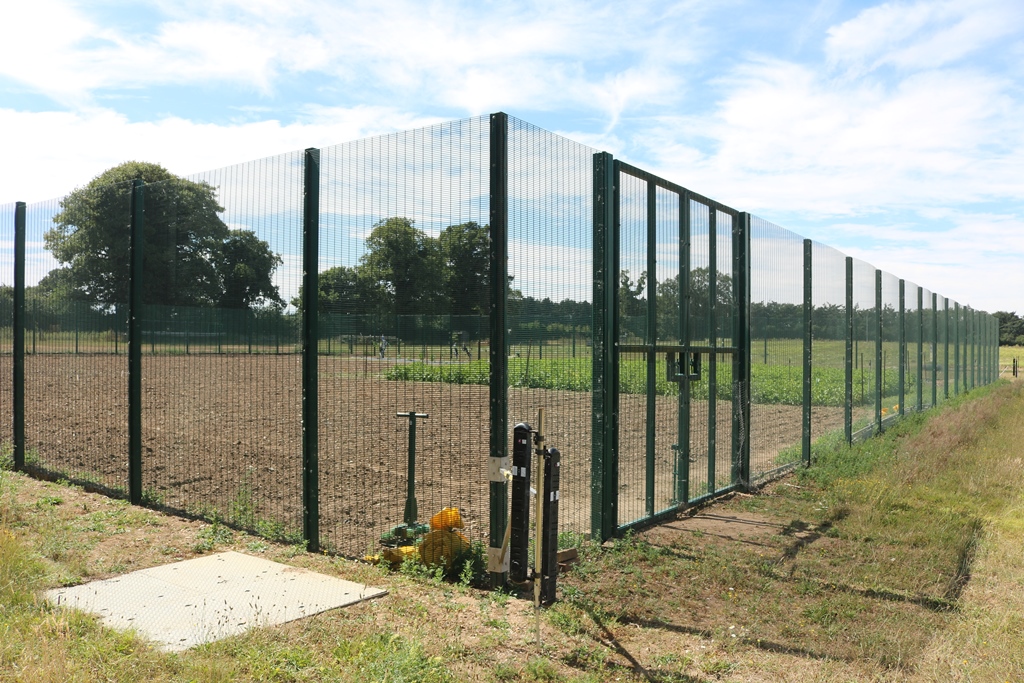
GMO field trials don’t require such high security, but they must be conducted to a level of regulation that’s currently above what’s required for conventional crops.
GMO field trials don’t require such high security, but they must be conducted to a level of regulation that’s currently above what’s required for conventional crops. That makes them prohibitively expensive or burdensome for many involved in wheat research, says Wendy.
“It means we can’t involve breeders in any elements of our research that involve NPBTs, and we’ve learned through DFW how important it is for commercial pull through to have this engagement right from the start. But scientifically, gene-edited material doesn’t need to be regulated differently from conventionally-bred plants.”
Cristobal explains that the mutations he and his team generate occur every day in the field and do not involve the introduction of foreign DNA. “The difference with a natural mutation or one that is chemical-induced is that our technique is precise – we know exactly where in the wheat genome the change has occurred. Typically, the technique just a few letters of DNA code in a plant that has several billion. There have been concerns for so-called ‘off-target’ effects, but we have a system to check for these, and a wheat seed typically has around 90 natural mutations with respect to its mother plant anyway,” he notes.
Through marker-assisted breeding, scientists now know the locations in the wheat genome responsible for a specific trait. The way in which DFW relates the phenotype – what’s seen in the field – with the genotype now allows the potential for traits to be edited direct to a wheat genome without having to make a cross that brings with it undesired genetic changes.
“In just one process, gene-editing delivers as near to an isogenic line as you can get,” he points out. “A farmer wouldn’t be interested in growing a landrace variety no matter how good its disease resistance or size of its grain, nor would they grow Paragon with that trait introduced. But they would grow Skyfall with an improved grain or better yellow rust profile. That’s why genotype independence is such a key point.”
Scientists currently work with varieties such as spring-sown Paragon, Cadenza and Fielder – a US milling wheat – because their genomes have been fully mapped and their phenotypes heavily researched. This makes them suitable templates for novel traits when working with breeders. But Cristobal wants to work directly with commercial varieties and involve farmers as well as breeders in his research.
“Under the current regulatory regime, the UK is falling behind on gene-editing, although this is not about leading the world. It’s about making use of a technology that can accelerate how we bring our discoveries to the field, and the shorter timescales will bring in farmers.
“Gene-editing is also relatively inexpensive if it’s regulated as conventional breeding. So unlike GM, we don’t need large multi-nationals to make a step change in how we grow wheat – there is potential for small-scale collaborations to bring forward niche ideas and innovations. This could not only lift the UK off its yield plateau, but provide solutions for the world for a more sustainable crop. We have the talent in plant science, breeding and farming to achieve this. All it needs is a science-based policy to govern its direction.”
What is a GMO?
This lies at the heart of the debate on NPBTs. Under EU law, any process that involves the introduction of foreign DNA or RNA classes the resulting product as GMO. But there are a number of ways through which a plant can undergo a genetic change:
Transgenesis is where DNA from another species has successfully been combined into the genome of the host plant. This confers a new trait, such as herbicide tolerance or longer shelf life. These organisms are universally classified as GM.
Cisgenesis is term used by some scientists who argue for light-touch regulation, where DNA is artificially transferred between organisms of the same species, such as from a wild relative to an elite potato variety to confer blight resistance. In Europe at least this is still classified as GM as nucleic acid sequences must be isolated and introduced using the same technologies that are used to produce transgenic organisms.
Mutagenesis is a change or edit in the plant genome that confers a new trait. Such mutations occur naturally every day, when a plant comes under stress, for example, or it can be induced through human intervention. A small change in the genome may switch off the activity of a particular gene which allows or inhibits a property, and it’s these phenotypical changes breeders have sought out for generations to progress their lines.
Is it natural?
For decades, scientists have induced mutagenesis to bring about new traits, using chemicals or radiation, and the Clearfield trait is an example. More recently, more precise gene-editing techniques such as CRISPR-Cas9 have been introduced. CRISPRs are short RNA sequences introduced into the host plant that recognise a specific stretch of genetic code. Cas9 enzymes partner these sequences and cut the host DNA at specific locations.
The cell tries to repair the damage, and that’s when the mutation occurs. By using different enzymes and techniques, researchers can deactivate or alter – edit – specific parts of the genome, thereby conferring traits. Scientists argue the genetic edits are simply a precise and predictable way of inducing a change that could have occurred naturally. But under EU law, the introduction of foreign RNA, even though the RNA is not present in the final plant material, classes current forms of CRISPR as GMO.